Scotts Contracting St.Louis Design Build Sustainable Building Contractor-providing diversified quality service at a fair price. For all of your remodeling, repairs, and maintenance needs.
Search This Blog
6.30.2010
Energy Use in the US Statts and Figures
How many renewable energy facilities covering how much area are required to meet the electrical energy demand of the United States? The following will identify some critical issues along with a possible solution, while demonstrating that renewable energy resource installations could be available to meet the required demand, should sufficient will be exerted to actually install. One of the major dilemmas facing the widespread implementation of renewable energy resources is resolution of how to distribute the newly installed resources. The existing grid is predicated on the use of very large centralized generation sources, e.g., dams, power plants; while most renewable energy, e.g., photovoltaic, wind, is very conducive for distributed generation.
The existing very large generators are large in the sense of the amount of power they produce per unit area. Renewable sources require much more land area for a comparable power production. A major benefit of this conundrum could be the installation of a large number of small generation sources at existing sites, e.g., houses, businesses, ranches, farms with no requirements to install additional distribution capacity. The downside is how to plan for the transfer of energy from where generated to where needed when the renewable energy generators are not firm, i.e., the amount generated is neither constant nor predictable. This is exacerbated by the financial consideration that nonrenewable generators are generally most efficient and cost effective when operated at full capacity. A model for solving the problem or more accurately, debugging the solution, is to initiate the widespread use of renewable energy generation in rural areas. Although eventually the largest market will be in urban areas, virtually all problems could be resolved on a small scale by implementation in rural areas first. Rural areas have a small fraction of the total population; however, this fraction is highly independent and well skilled in solving problems. Unfortunately, this is also the segment of the population that has the least disposable income to invest in anything, much less energy with a long term payback. Thus, some sort of cash flow assistance will be required, noting tax credits are of little benefit to those whose income is not sufficient to pay much in taxes. In order to understand the magnitude of the task, one must consider how much electrical energy is going to be required to be converted from nonrenewable to renewable sources. The United States consumes ~ 4.1 trillion-kWh per year (4.1x1012 kWh/year), note this does not include fossil fuel energy consumption for transportation, heating, and other uses. Since a significant fraction is required for industrial use, which requires large concentrated sources, e.g., existing power plant dams will still be operational for 100 or more years depending on location, one could then reasonably expect the want to generate ~ 3 trillion-kWh per year with renewable sources. There are 5 primary sources of renewable energy generators in operation today; four sources can be used for large commercial (e.g., utility scale, light industry, or towns) generation and three sources that are primarily for residential use. Depending on size of the installation, two of the sources can be in either category.
For wind, assume that 0.99 trillion-kWh/year are produced by commercial size wind generators and the rest with residential. Assume that each 5 MW wind generator operates 12 hours per day for 300 days per year (allowing time for maintenance and variations in wind velocity and duration). Each wind generator then provides 18 Mega-kWh/year. Thus, approximately commercial 55,000 wind generators are needed. If each wind generator occupies ~ 1 square mile, then ~ 55,000 square miles are needed, noting that almost all the land near a wind generator can be used for ranching or farming purposes. This represents a small fraction of the land under cultivation in the western USA, where much of the wind resources are. Also, wind generators can be place off-shore. For residential wind generators, assume that each 15 kW windmill operates 6 hours per day for 250 days per year (allowing for conversion efficiency, time for maintenance, and variations in wind velocity and duration). Each wind generator then provides 22.5 kilo-kWh/year. Thus, approximately residential 450,000 wind generators are required, which is significantly lower than the total number of small businesses, farms, ranches, and rural residences in the USA. With larger residential windmills, especially for farms and ranches, not so many windmills would be required. A 25 to 50 kW windmill is much more appropriate for farm or ranch use, noting some farms that use well irrigation would need several wind generators or larger, e.g., 150-200 kW wind generators. For residential PV, assume a module conversion efficiency of 15% from the nominal solar radiance of 1000 W/m2. Assume a DC to AC conversion efficiency of 85% and operation for 6 hours per day for 300 days per year (allowing for variations for systems installed at a wide variety of locations). Thus each m2 of solar module area will produce 230 kWh/year. In order to generate, 0.5 trillion-kWh/year, there needs to be ~2,175,000,000 m2 of PV modules. This is about 840 square miles of solar PV modules, smaller than most Western state counties. Assuming that the majority, say 1,500,000,000 m2, are directly used on single family dwellings, with the availability of 75 m2 per dwelling (still allowing room for solar hot water heating collectors on the south facing roof), then ~20,000,000 homes are necessary. The remaining PV generation (~ 0.16 trillion-kWh/year) would come from commercial PV facilities. Assuming a capacity of 200 kW operating 8 hours per day (use of at least ground mount single-axis trackers) for 320 days per year (allowing for variations for systems installed at a wide variety of locations) each location would generate 512,000 kWh/year. There would need to be at least 312,500 such installations, with each installation having about 1,570 m2 of PV modules and assuming an area efficiency of 10% (for trackers and mounting) so occupying ~ 4 acres. For solar thermal generation, assume each 100 MW of power capacity requires 1000 acres, including all support structures. Since solar thermal requires significant water usage for cooling (up to 1000 acre-feet/year per 100 MW), not all locations are suitable. Assuming a 500 MW plant produces 8 hours per day for 300 days per year, each location produces 1.2 billion-kWh/year. For the 1 trillion-kWh/year, then ~850 plants, occupying 4.25 million acres or 6,640 square miles (the size of larger Western state counties) All of these estimations are just that, estimations; however, the numbers clearly show that renewable energy resources can provide the majority of the electrical energy needs of the USA. As renewable energy resources are installed, no new fossil fuel power plants need be built. Eventually, all fossil fuel plants can be allowed to retire, starting with the least efficient first. The transition cannot be smooth, since both nonrenewable and renewable energy sources are only available in discrete units; however, by starting with implementation in rural areas the methods and techniques can be fully developed, which will ease large scale implementation in urban areas. Reference |
6.29.2010
New System, Algae Cleans Water & Fertilizes
act as slow-release fertilizer
seedlings could thrive on an organic fertilizer
management of the cycle of nitrogen and phosphorus
capture costs of around $5 to $6 per pound of nitrogen and $25 per pound of phosphorus
The system is practicable now
Testing: could clean up runoff that has already made it into the water system
Tags
- Science, Jeremy Hsu, agriculture, algae, environment, farming, fertilizer, water, water purification
In New System, Algae Cleans Water, Then Transforms into Organic Fertilizer
- The algae systems can capture most of the phosphorus and nitrogen in runoff
- By Jeremy Hsu Posted 05.07.2010 at 3:57 pm 7 Comments
Nature's Green Cleaner Air-dried algae (shown above) from an algal turf scrubber captured most of the nitrogen and phosphorus in the manure. USDA/Edwin Remsburg
Algal blooms that feed on nutrient-rich manure and fertilizer runoff can deplete oxygen in the water when they die, creating inhospitable dead zones -- but the same green scum might also serve as a preventive solution upstream. A microbiologist with the U.S. Agricultural Research Service used algae to recover almost 100 percent of nitrogen and phosphorus nutrients from manure, and suggested that the dried-out algae can then act as slow-release fertilizer for farms.
The solution offers better management of the cycle of nitrogen and phosphorus nutrients which plants depend on. Experiments have shown that algae can capture 60 to 90 percent of nitrogen and 70 to 100 percent of phosphorus from a mixture of manure and fresh water, as proved by the U.S. Department of Agriculture (USDA) on four dairy farms.
The system is practicable now. Farmers would have to set up algal turf scrubber (ATS) raceways covered with nylon netting to serve as a platform for algae to grow upon. The capture costs of around $5 to $6 per pound of nitrogen and $25 per pound of phosphorus is about the same as other manure-management practices.
But Walter Mulbry, the USDA microbiologist, also showed that corn and cucumber seedlings could thrive on an organic fertilizer made from the dried-out algae. That might allow farmers to recoup even more of the costs from the ATS system, or perhaps turn a profit if the price is right.
Mulbry has already begun another study to see whether fertilizer made from chicken and poultry litter can also benefit from the algae cleanup system. And he has also begun studying whether the ATS systems can remove nitrogen and phosphorus from estuaries that flow into the Chesapeake Bay, so that they could clean up runoff that has already made it into the water system.
Related Articles
| |
| |
|
Algae's versatility has already won over scientists who see it as the biofuel of the future, and the tiny plant organisms have also been proving their worth in scrubbing carbon dioxide and nitrous gas from industrial smokestacks. A company called Algenol has even looked to using algae-derived plastic as a replacement for petroleum-derived plastic.
Even the U.S. Department of Energy and various branches of the U.S. military have begun seriously exploring algae-derived solutions. If that doesn't entirely ensure a clean future, it at least suggests a future with a scummy color palette ranging from pale to bright green.
[USDA]
- Brought to you by: Scotts Contracting GREEN BUILDER_St Louis "Renewable Energy" Missouri http://www.stlouisrenewableenergy.com, contact scotty@stlouisrenewableenergy.com for additional information
Great News in Wind Turbine Development.
I happily bring the following article to my readers.
Scotts Contracting offers Wind Power Systems and the Required Installation and Electric Hook-Up.
contact scottscontracting@gmail.com to schedule your free Green Site Evaluation.
Massive Wind Turbine Survives Pummeling By Equally Massive Testing Machine
- By Rebecca Boyle Posted 05.27.2010 at 9:30 am 4 Comments
Massive Turbine Test Samsung's 90-ton, 2.5-megawatt wind turbine drive train meets the National Wind Technology Center's 2.5-megawatt dynamometer. Rob Wallen, National Renewable Energy Laboratory
Wind turbines of the future will be hulking behemoths, each capable of producing multiple megawatts of power. But before they're installed in wind farms, manufacturers need to be sure they are built to last. To this end, a monstrous 2.5-MW turbine--one of the world's largest--just survived an equally big test.
The 2.5-megawatt dynamometer at the National Renewable Energy Laboratory blasted the turbine's drive train, built by Samsung, with 12.6 million inch-pounds of torque, the energy lab says.
In other words, the turbine drive train went through years of wear and tear in about two months. It was the largest full-scale dynamometer test of a wind turbine ever done in the United States, NREL says.
The dynamometer — a cool name for a machine that measures force and torque — has a 3,550-horsepower electric motor coupled to a three-stage epicyclic gearbox, according to NREL. It can produce speeds up to 30 revolutions per minute, meaning it can simulate anything from a slight breeze to a full-force gale.
Related Articles
| |
| |
|
Tags
- Technology, Rebecca Boyle, environment, renewable energy, vertical wind turbine, wind power, wind turbines
- Computer models simulate the tower, rotor and turbine blades, and other models calculate what the main shaft torque should be, depending on weather conditions. The shaft therefore responds to various wind conditions just like it would in the field, NREL says.
-
- Special Turbine Delivery: NREL technicians unload a 90-ton Samsung wind turbine drive train after it made the trip on semi-trailers from Houston to NREL's National Wind Technology Center. Rob Wallen, National Renewable Energy Laboratory
Samsung has a 2.5-MW turbine in operation in Texas, but it's never been tested above 600 kilowatts. Samsung wanted to take its drive shaft out for a spin, so the company shipped the 185,000-pound device from Houston to Golden, Colo., using a gigantic 185-foot-long, 19-axle rig.
All this bigness is peanuts compared to future wind turbines, however — NREL is already building a 5-MW dynamometer, which would be capable of testing the next generation of huge wind turbines.
--
Scott's Contracting
scottscontracting@gmail.com
http://www.stlouisrenewableenergy.blogspot.com
http://www.stlouisrenewableenergy.com
scotty@stlouisrenewableenergy.com
Solar Energy-Our Government, Govt Fees Hinder Solar Development
Solar Energy-Our Government, Govt Fees Hinder Solar Development
this article is somewhat of a follow up article post: http://stlouisrenewableenergy.blogspot.com/2010/06/energy-in-our-future-where-will-it-come.html. Where I posted: "c) Politics within the USA will Keep the Renewable Energy Resources from becoming Main Stream and affordable to the masses."I urge everyone to Contact your Legislative Department and let them know you approve Solar Energy Production. Feel Free to utilize the following Link.sign the letter now.
America faces unprecedented economic, national security and environmental challenges. The solution – transition to clean, renewable energy. Join our movement of more than 5 million calling for clean energy and climate policies that will create millions of jobs, make us energy independent and solve the climate crisis.
sign the letter now.Tags
Government Fees Could Be Hindering the Rollout of American Solar Power
- A bureaucracy built for oil and gas leasing punishes the most efficient technology
- By Clay Dillow Posted 06.17.2010 at 2:17 pm 43 Comments
Solar One's Mojave Desert Solar Plant U.S. Department of Energy
Given the way the government is beating up on a certain foreign oil company this morning, it's easy to think perhaps this is the impetus America needs to align government, industry, and popular sentiment toward developing home-grown renewable energy sources. But a look at the charges the Bureau of Land Management levies against solar power projects on public lands tells a different tale. In fact, it seems the more efficient your power plant, the more the BLM wants you to pony up.
The government justifiably charges private businesses rent if they want to operate solar or wind arrays on public lands. But on top of that there is a compicated fee structure -- the "megawatt capacity fee" -- that drives the total rent as high as twice the market value for comparable private land. The BLM -- again, justifiably -- says its just trying to get taxpayers something back for private enterprise that benefits from the land they own. But the way the fees are structured is somewhat suspect.
Related Articles
| |
| |
|
The BLM is essentially charging more efficient technologies more in megawatt capacity fees. According to NYT's Green Blog, a photovoltaic plant that deploys big PV panels has to pay $5,256 per megawatt. But more efficient solar thermal plants that use solar energy to turn a steam-driven turbine are hit with a $6,570 fee. If either technology is coupled with an energy storage system that delivers power even when the sun doesn't shine -- a key component to making solar power more than just a secondary energy resource -- the fee takes a flying leap to $7,884 per megawatt.
If you're generating 550 megawatts, like First Solar's Riverside County, Calif., PV array is expected to, that's a lot of money, and the consumer has to pay that. We're more scientists than economists over here, but that fee structure seems like it creates a vast disincentive for companies to deploy more efficient, better solar technologies. It's a consequence of trying to adapt archaic oil and gas leasing models to new energy paradigms, and frankly it seems at odds with our stated goal -- and I'm talking directly to President Obama and DOE chief Stephen Chu here -- of developing renewable energy resources right here at home.
Public lands belong to taxpayers and indeed private enterprise should pay fair market value to lease them, but saddling these projects with outrageous costs pushes up the price of solar energy, keeping carbon fuels in their current position as market darling. Weaning ourselves from foreign oil -- and eventually all oil -- seems a far better public reward than a monetary benefit paid to the public coffers.
Scotts Contracting offers Free Green Site Evaluations for the Solar Powered Energy in your Home and Business- click here to email Scotts Contracting
June 29-New Solar Cell Technologies-
thin-film, flexible copper-indium-deselenide solar cells
cheap to produce
tuff and sturdy enough to withstand the stresses of the battlefield.
That means flexibility, durability, and efficiency never before seen from commercial solar technology.
Tags
- Technology, Clay Dillow, battlefields, energy, environment, green tech, military, photovoltaics, renewable energy, solar cells, solar power
To Power Future Battles, DARPA Wants Combat-Tough Solar Cells
- By Clay Dillow Posted 06.23.2010 at 10:00 am 2 Comments
Making Better Solar- Researchers work on a sheet of flexible solar cells at the University of Delaware's Institute of Energy Conversion. University of Deleware Institute of Energy Conversion
In recent years, the U.S. military has been making small strides toward a greener energy standard – the Navy wants to create a green strike group by 2012, while the Air Force has been testing biofuels in its aircraft. But for troops on the ground relying increasingly on electronic devices, solar is the way forward. With that in mind, DARPA has assembled an industry-academic team of photovoltaic leaders to create the next generation of battle-ready solar cells that achieve 20 percent conversion while standing up to harsh combat conditions.
The $3.8 million dollar, 54-month Low-Cost Lightweight Portable Photovoltaics program (PoP) includes several private PV companies and will be led on the academic side by the University of Delaware's Institute of Energy Conversion (IEC) with the goal of demonstrating solar cells that are not only cheap to produce, but sturdy enough to withstand the stresses of the battlefield. That means flexibility, durability, and efficiency never before seen from commercial solar technology.
|
|
|
|
|
|
To do so, the research team will push thin-film, flexible copper-indium-deselenide solar cells to their limits, tapping the technology portfolios of at least four different industry partners to meet DARPA's goals, which – per usual – are lofty even given the four-and-a-half year time frame. By comparison, commercial solar panels range from 5-20% efficiency, but flexible thin-films generally average somewhere between 7-11% efficiency, and they're nowhere close to combat-ready.
To make the leap, the team will obviously have to focus on the dual goals of upping efficiency while turning the delicate into the durable. That's going to take some serious innovating in materials and PV tech, but the good news – as is often the case with DARPA projects – is that advances in the technology should eventually trickle on down to civilian tech, giving all of us tough, portable solar powered devices to abuse.
--
Scott's Contracting
scottscontracting@gmail.com
http://www.stlouisrenewableenergy.blogspot.com
http://www.stlouisrenewableenergy.com
scotty@stlouisrenewableenergy.com
Carbon Definition, Investing, Carbon Foot Print, and the Top 9 Carbon-Neutral Communities
What is Carbon? Wikipedia Defines it as: Carbon is the chemical element with symbol C and atomic number 6. As a member of group 14 on the periodic table, it is nonmetallic and tetravalent—making four electrons available to form covalent chemical bonds. There are three naturally occurring isotopes, with 12C and 13C being stable, while 14C is radioactive, decaying with a half-life of about 5730 years.[9] Carbon is one of the few elements known since antiquity.[10][11] The name "carbon" comes from Latin language carbo, coal.
Additional Carbon Info, Carbon Footprints and Carbon Investing- What You Should Know Click Here!
The following Article gives the stats of the World's Carbon-Neutral Communities.
Nine of the World's Most Promising Carbon-Neutral Communities
- In the global race to reduce carbon emissions, these eco-minded communities, from Kansas to the Maldives, lead the pack. Here's how they're making their carbon footprints disappear
- By Patrick Di Justo Posted 06.17.2010 at 10:18 am 37 Comments
- Building a Carbon Neutral Paradise eBoy
"Carbon neutral" sounds pretty straightforward—simply remove as much carbon dioxide from the atmosphere as you put in. The trouble is, civilization began emitting CO2 when humans burned the first lump of coal about 4,000 years ago.
Ever since, we've been digging up carbon in the form of fossil fuels and sending it skyward. Today the fraction of carbon dioxide in the atmosphere is about 385 parts per million, roughly 30 ppm above the level deemed environmentally sustainable by many scientists and climate experts. If we continue emitting at our current rate, that number will climb to 450 ppm by 2050, putting the planet at serious risk of runaway climate change, with heat-trapping gases causing unprecedented warming. After that, things get pretty scary: Coastlines flood, species disappear, droughts get longer, crops become harder to grow, and people in general become hungrier, thirstier and angrier.
But it's not too late to reverse course. Fortunately, thousands of communities, cities and even whole countries are pursuing real carbon neutrality, enacting legitimate plans to mitigate their greenhouse emissions and, eventually, bring atmospheric CO2 concentrations back to safe levels. Here, we've spotlighted some of the most inspiring. Denmark's Samsø Island, for example, is no mere engineering fantasy. It's already balanced its carbon footprint by adopting wind and solar power and is now working toward offsetting more carbon than it emits. Masdar City in arid Abu Dhabi, meanwhile, is proving that even oil-rich regions with outsize carbon footprints can wean themselves off the black gold. More important, each of these projects is employing energy and conservation strategies well within the reach of any developed nation that really wants to implement them.
These impressive efforts, though, reveal just how far we have to go. The average Maldivian uses so little fossil fuel that the global benefits of a carbon-free Maldives can be undone by just five days of average population growth in the U.S.
Ultimately, carbon neutrality demands a global effort. That means communities from New York to New Delhi must transform—in effect, becoming perfectly balanced biomes: lots of activity, lots of creation and destruction, but without all the CO2 emissions that typically go with it. In other words, carbon neutrality requires a complete rethinking of how we have lived since the Bronze Age. Impossible? There are at least nine ecotopias that are proving otherwise.
Best U.S. Project
Greensburg, Kansas
Size: 1.5 square miles
Population: 900
Annual carbon emissions per person: 22 tons
Annual amount to be offset: 19,800 tons
Equivalent to: 825,000 trees planted
Carbon-neutral by: 2017
On May 4, 2007, a tornado ripped through central Kansas. When it was over, nearly 95 percent of the small town of Greensburg was destroyed. Turning tragedy into opportunity, the community decided to rebuild itself as a model green town, powered by a mix of geothermal, solar and wind. A few of the city buildings even power themselves with their own wind and solar generators, and a 10-turbine wind farm outside of town went online in March.
Holiest
Vatican City
Size: 109 acres
Population: 826
Annual carbon emissions per person: 9 tons
Annual amount to be offset: 7,434 tons
Estimated cost: undisclosed
Carbon-neutral by: undisclosed
In recent years, the Catholic Church has been more active on environmental issues, urging people to be better stewards of the Earth while holding itself up as a model. In 2007, Pope Benedict XVI, dubbed "the green pope" for his environmental initiatives, announced his intention to make the Vatican Europe's first carbon-neutral state, starting with solar panels on the Vatican's auditorium that produce 300,000 kilowatt-hours a year, coupled with extensive efforts to conserve energy.
Fastest Transformation
SamsØ Island, Denmark
Size: 44 square miles
Population: 4,000
Annual carbon emissions per person: 12 tons
Annual amount to be offset: 48,000 tons
Number of wind turbines: 21
Estimated cost: $39 million
Carbon-neutral by: 2007
Remarkably, the Danish island community of Samsø has become carbon-neutral without any tax breaks, grants or other benefits. Its motivation? Civic pride. Thanks to conservation, wind power and peer pressure, the island has transformed from a consumer of coal and oil into an exporter of alternative energy. It now generates 10 percent more energy than it uses.
Most Urgent
Maldives Size: 115 square miles Population: 396,334 Annual carbon emissions per person: 2 tons Annual amount to be offset: 792,668 tons Estimated cost: $1.1 billion Carbon-neutral by: 2020
If ever a country had a stake in carbon neutrality, it's the Maldives—a sea-level rise of just a few inches could put most of this island nation underwater. By investing tourism dollars in energy infrastructure and offsets, Maldivians hope to set an example of carbon sustainability that the world can follow. If that fails, they're considering squirreling away enough money to buy a patch of high ground in India or Australia.
Most Ambitious
Costa Rica Size: 19,730 square miles Population: 4.25 million Annual carbon emissions per person: 2 tons Annual amount to be offset: 8.5 million tons Equivalent to: 354 million trees planted Estimated cost: $10 billion Carbon-neutral by: 2021
In 2007 the Costa Rican government declared that the country would be carbon-neutral by 2021, in time for its 200th birthday. Three years later, it's virtually there. It already produces 90 percent of its electricity from renewable sources, mostly hydropower, wind and geothermal. Next up, it will add solar to the mix, introduce electric trains and buses, move to clean biodiesel and bio-ethanol fuel for cars, and help reforest its jungles.
Smallest
BedZED, London Size: 4.45 acres Population: 220 Annual carbon emissions per person: 11 tons Annual amount to be offset: 2,420 tons Estimated cost: $22 million Carbon-neutral by: 2002
Every square inch of London's Beddington Zero Energy Development is designed for sustainable living. Each of its 100 homes and 15 apartments was built from local materials (to limit haulage) and features triple-glazed windows, solar panels, a biofuel boiler, sustainable insulation and low-energy appliances, with eye-level electric and water meters in all the kitchens to remind residents why they're living there in the first place
Most Resourceful
Dockside Green, B.C., Canada Size: 15 acres Population: 2,500 Annual carbon emissions per person: 21 tons Annual amount to be offset: 52,500 tons Equivalent to: 9,100 cars removed Estimated cost: $600 million Carbon-neutral by: 2011
All of the buildings populating this small development on Vancouver Island are certified green by the internationally recognized U.S. Green Building Council. Eco-features include a "bio boiler" that runs on gas generated from wood refuse, efficient lighting and electrical appliances, and a graywater recycling system that uses treated sewage water to flush toilets.
Most Down-to-Earth
Moreland, Australia Size: 19.7 square miles Population: 149,122 Annual carbon emissions per person: 23 tons Annual amount to be offset: 3.4 million tons Equivalent to: 595,000 cars removed Carbon-neutral by: 2030
Australia's per-capita carbon footprint rivals that of the U.S. So how does this bedroom community of Melbourne intend to shrink it to zero? Through education and energy audits, and by providing seed money to establish local renewable-energy systems such as solar and wind. No whiz-bang tech; just a slow and steady race toward smart conservation and renewable energy.
-=-=-=-=-=-=-=-=
--
Scott's Contracting
scottscontracting@gmail.com
http://www.stlouisrenewableenergy.blogspot.com
http://www.stlouisrenewableenergy.com
scotty@stlouisrenewableenergy.com
Green Buildings and Our Future Part 4
The Future of Green Architecture: A Live-In Power Plant
- This concept skyscraper could generate enough energy to power 4,000 homes
- By Suzanne LeBarre Posted 06.03.2010
-
-
At first glance, the plans for the 10MW Tower have all the trappings of pre-crash Dubai: the improbable height, the flashy facade, the swagger of a newbie in a crowded skyline. On closer inspection, however, it's an eco-machine. The A-shaped, 1,969-foot concept skyscraper is designed to turn out as much as 10 times the energy it needs, enough to power up to 4,000 nearby homes.
- Reflective: The facade directs light to a power-producing salt-cooker. Courtesy Robert Ferry/Studied Impact Design
Three separate systems make it work. First, a five-megawatt wind turbine in the hollow of the "A" generates energy in the powerful and unpredictable desert gusts. Second, mirrors dot the slanted, south-facing facade, beaming light to a molten-salt-filled collector that hangs off the building like an ultra-tall street lamp. Cooked to 932ºF, the liquefied salt transfers heat to a convection loop that runs a three-megawatt steam turbine. Finally, a two-megawatt solar updraft tower produces additional energy in clear weather. Sunlight warms air in a two-foot-wide gap that runs the length of the southern face. The airflow from rising heat powers an internal wind turbine.
If it were built (at an estimated cost of $400 million), 10MW could pay off its energy debt in 20 years. Extra juice feeds the municipal grid, and other sources in the area would adjust for the tower's output. The building could house offices or residences or both, says designer Robert Ferry, 35, who helms the Dubai architecture firm Studied Impact with his wife, Elizabeth Monoian. The pair became interested in energy-generating skyscrapers on moving to the United Arab Emirates, where there are superstructures in spades but few that are any greener than their brochures. With the 10MW Tower, they hope to someday create a power plant you can live in. It may sound fantastic, but, Ferry says, "it's only a matter of time before something like this is built."
- Revolutionary: A five-megawatt turbine contributes to the building's annual output of 20,000 megawatt-hours. Courtesy Robert Ferry/Studied Impact Design
Scotts Contracting offers Green Building Services for the St Louis Metro Area Click here to email Scotty to schedule a Free Green Site Evaluation
--
Scott's Contracting
http://www.stlouisrenewableenergy.blogspot.com
Green Buildings and Our Future Part 3
A rising Sea Level will make the following article a clue to where we will live in our Near Future.
The Future of Green Architecture: A Floating Museum
- By Suzanne LeBarre Posted 06.21.2010 at 11:56 am 15 Comments
-
-
- Physalia A museum, nightclub and filtration system, Physalia uses its hull and rooftop plants to scrub away pollution. Courtesy Vincent Callebaut Architecture
Physalia is half-boat, half-building, and all green. This mammoth aluminum concept by Belgian architect Vincent Callebaut is meant to travel Europe's rivers, making filthy water drinkable. At the same time, the ship generates more energy than it uses.
A coat of titanium dioxide paint brushed onto the silvery shell will neutralize pollution by absorbing ultraviolet rays, enabling a chemical reaction that decomposes organic and inorganic toxins. (It's the same technology used in certain high-tech concrete that breaks down airborne particulates.) As the vessel whips along, purifying waterways, it can draw on both solar and hydro power. Turbines under the hull transform water movement into electricity, and rooftop photovoltaic cells harness energy from the sun. The roof doubles as a nursery, whose carefully selected plants help filter river gunk, whether from the Thames, Rhine or Euphrates.
But Physalia isn't just designed to be a working ship. The vessel will also be a floating museum of sorts. Scientists who study aquatic ecosystems can hole up in the dedicated "Earth garden" lab, and tourists can visit temporary exhibits in a "water garden" or settle into a submerged lounge that could easily pass for a London nightclub. Callebaut, 33, dreamed up the idea after last year's United Nations Climate Change Conference in Copenhagen shone a long-overdue spotlight on global water issues. He has some lofty terms for his project: It's a "nomadic hydrodynamic laboratory," a "fragment of living earth," and a "floating agora" on a "geopolitical scale." Others might just call it a cool idea.
-=-=-=-=-=-=-=-=-=-=-=-=-=-=-
Scotts Contracting offers Green Building Services for the St Louis Metro Area
Click here to email Scotty to schedule a Free Green Site Evaluation
--
Scott's Contracting
scottscontracting@gmail.com
http://www.stlouisrenewableenergy.blogspot.com
http://www.stlouisrenewableenergy.com
scotty@stlouisrenewableenergy.com
Green Buildings and Our Future Part 2
The following Article Points out the ways we can save and utilize water more efficiently in our Homes. I think back to my college Drafting Classes and the Instructor's thoughts on water use in the Home. What I once thought was far-fetched ramblings of an Old Foggey and his ideas of recycling water- for use in the Home. I do now believe will become a reality as Science & Green Building Technologies Improve.
The Green Dream: Going Gray, Saving Blue
- A graywater system uses shower and sink runoff to flush the toilets. Plus: four more ways to save water at home
- By John B. Carnett Posted 06.24.2010
-
Green Dream: The Specs House: 3,500-square-foot, four-bedroom contemporary Location: Greenwich, N.Y. Project: Install graywater recycling Cost: About $2,600 ($1,400 for the system; $1,200 for plumbing) Time to install: 2 hours Eco-advantage: Uses household runoff for toilets, saving water and work for the septic system Peter Bollinger
Just because residential water is cheap and plentiful here in upstate New York is no reason to waste it, and the average household does plenty of wasting: A single flush consumes three to seven gallons of water. Inefficient toilets and long showers are two of the biggest water wasters, together accounting for more than 40 percent of the 350 gallons of water used daily in a typical American home. But my eco-home is anything but typical—its graywater recycling system can save at least 110 gallons a day.
Graywater refers to the runoff from sinks, showers and washing machines (as opposed to blackwater, which contains solid waste). With some basic plumbing and a storage tank, it's easy to recycle that water to flush my house's four toilets. By using the water twice, I'll also save wear on my septic system.
The setup is pretty straightforward. Water from the bathroom sinks and showers goes through a chlorination filter and into a holding tank, where it can be pumped to the toilets. (I'm skipping the washing machine and kitchen sinks, since they require additional filtering and I'll recycle plenty of water from the bathrooms alone.) It's not difficult to DIY, but the central challenge is monitoring and controlling the level of chlorine in the storage tank. Too little, and you'll get bacteria in the tank. Too much, and it will kill the bacteria your septic system needs. So I'm using a new setup from a company called Blue Eco Systems that funnels water through a chlorinator to carefully control how much chlorine goes in. Carbon filters on the overflow and bypass lines prevent chlorine from getting back into the septic tank and the toilets, lest my bathroom smell like a swimming pool.
The system uses a pair of 25-gallon tanks—enough for my family of four—but I can easily expand it with more tanks if we have more kids or the in-laws move in.
How It Works: Green Dream: Peter Bollinger
How the Green Dream's Plumbing Works
Fresh water in: If there's not enough graywater in the tanks for a flush, the system pulls in regular street water.
Graywater in: Water coming from bathroom sinks and showers
Graywater to Toilets
Carbon filters: Remove the chlorine from the water before it reaches your toilet or septic system so it doesn't kill the bacteria the septic system needs
Chlorinator: Cleans the graywater to prevent bacterial growth in the tank
Vent
Overflow: Carries extra water out to the septic system so the tank doesn't overflow
Pump: Sends the water from the tank up to the toilet tanks
Flow sensor:If the system detects no water flow in 22 hours, it dumps the contents of the tank so it doesn't sit long enough for any remaining bacteria to grow.
Four More Ways to Save Water at Home
Sprinkler Shutoff A broken sprinkler can waste 100 gallons in 10 minutes when the irrigation system kicks on in the early-morning hours. This automatic shutoff valve prevents waste by holding water in the irrigation tubes if the sprinkler head is broken, saving about 65 percent more water than a system without one. From $4; dry-planet.com
Greener Grass Most grasses in America are indigenous to Europe, so they need more water and maintenance. The University of Nebraska is developing a new type of buffalo grass, native to the U.S., that will require between 40 and 75 percent less water than foreign species like blue grass or fescue. It will be available next January. From 50 cents per sod plug; toddvalleyfarms.com
Low-Flow faucet Install Niagara Conservation's simple aerator in your bathroom, and choose among three flow rates—0.5, 1.0 or 1.5 gallons per minute—depending on your task. All three settings use less water than the standard 2.2-gpm fixture, and the lower two even best the EPA's 1.5-gpm high-efficiency faucets. $11.50; niagaraconservation.com
John B. Carnett, PopSci's staff photographer, is using the latest green technology to build his dream home. Visit popsci.com/greendream for John's blog.
-=-=-=-=-=-=-=-=-=-=-=-=-=-=-
Scotts Contracting offers Green Building Services for the St Louis Metro Area
Click here to email Scotty to schedule a Free Green Site Evaluation
-- Scott's Contracting scottscontracting@gmail.com http://www.stlouisrenewableenergy.blogspot.com http://www.stlouisrenewableenergy.com scotty@stlouisrenewableenergy.comGreen Building’s and Our Future -Part 1
Sky's the Limit in the Latest Green Building Ideas as the following Article points out. A glimpse of our Future Cities, Travel and Commute for year: 2160.
Environmental Visionaries: The Urban Remodeler
- It would be easy to dismiss Mitchell Joachim's fantastical vision for ecological supercities, with their flocks of jetpacks and mass-transit blimps that look like flying monster jellyfish, as science fiction—if he wasn't actually building them. By John Bradley Posted 06.23.2010
-
Mitchell Joachim's Eco-City Nick Kaloterakis
Architect Mitchell Joachim points out, frequently and without prompting, that his futuristic proposals are always based on existing technologies. No wonder he feels the need to say it. Consider some of his ideas: jetpacks tethered together in swarms, houses grown from living trees, low-altitude blimps prowling New York City with chairs hanging below them for pedestrians to hop on and off (24/7 ski lifts on Broadway!), and WALL-E-like machines that erect buildings and bridges from recycled waste.
For Joachim, a 39-year-old professor of architecture at New York University, with graduate degrees from MIT, Harvard and Columbia, these concepts aren't Hollywood fluff but designs that could come to life today. Take his concept for waste-building machines, which he calls Rapid Re(f)use. Instead of the cubes of cardboard, plastic or steel that current recycling balers produce, Joachim's robots would grind and compress waste into I-beams, cruciform columns or even furniture components. The structures would be pressed or melted into shape or wrapped with metal bands, which is what recycling plants do now. All that would change is the shape—like switching the mold on a Play-Doh press, but on an industrial scale. "We could do it yesterday," Joachim insists.
His vision falls under the banner of Terreform ONE, a nonprofit design collective that Joachim co-founded to explore sustainable, fully integrated urban planning. If the same people who design the roads also design the cars, he says, and the same people who create the suburbs also plan for ways to feed and transport residents, our cities will become healthier, friendlier and more sustainable.
The group imagines how future cities might best serve their citizens on a large scale and then experiments with the small-scale materials and designs that it would take to make it happen. To this end, Terreform ONE hosts TerreFarm, an annual summer gathering of architects and scientists who develop new urban farming techniques. For several weeks this summer, TerreFarm will convert a Brooklyn rooftop into a testbed for modular growing methods, designs for maximizing available sunlight, and ultra-lightweight soil mixes essential for rooftop gardens. They will also build full wall sections of Joachim's Fab Tree Hab, his proposal to create "living" homes by grafting trees together around scaffolds and growing them on-site.
Joachim's other plans tend to focus on mobility, since transportation both shapes and is shaped by urban design. In his vision, individual cars would be replaced by car-share systems that function like luggage carts at an airport. Pay, step into a smart car that communicates with the city grid, drive to your destination, and leave the car there. The cars would have soft, springy exteriors, inflatable protective bladders and transparent foil, which would enable them to bump together as they traveled in flocks. "The idea of sharp metal boxes is just done," Joachim says. "We design cars with the principle that no one would ever die in a car accident again."
Joachim's blimps would move like trolley cars. Their routes would be set by funicular cables, and they would float slowly enough that pedestrians would be able to hop on and off hanging chairs dangling above the ground. Unlike a trolley car, though, the blimps would also be able to cross rivers, gorges and other geographic features without bridges.
His jetpack designs are not the retro-futuristic rocket belts of the 1960s but more like the lightweight ducted-fan jetpacks scheduled to go on sale later this year from Martin Aircraft Company. For efficient commuting, Joachim's jetpacks—soft and flexible like his cars—would be towed in flocks by a plane or blimp. "Bump and glide," as Joachim describes it. From there, individuals could break off, power up, and fly safely to their homes or offices—like subways in the sky. "It's hard to find people who don't want to be moving around in jetpacks in 20 years," he says. "As an architect, then, I'm responsible for thinking about what the implication of the jetpack is on the future city."
Joachim's willingness to forgo lucrative commercial projects in favor of running a nonprofit dedicated to the reimagining of a future he won't even be around for is, say his colleagues, exactly what makes him so vital. Traditionally, "cities are built incrementally by real-estate interests," says Richard Sommer, the dean of architecture at the University of Toronto. "What's important about Mitchell's work is that he [takes] a visionary approach."
The vision part involves looking 150 years down the road and planning for how cities will have to operate within the environment if civilization is to endure. Even if the technologies exist today, Joachim says, no one can change the city tomorrow. "Once we heard about cellphones, it was about seven years before we started dropping the landline," he says. "It took about 15 years before you could buy a hybrid car on every lot. It takes around 40 years to produce a large shift in the way buildings are constructed. Entire cities? It's 100, 150 years."
In the meantime, Joachim is busy producing the stuff that will get us there, whether it's growing living walls, planting organic lettuce on urban rooftops, or making sure that when your grandkids are ready for their first jetpacks, their cities will be too.
-=-=-=-=-=-=-=-=-=-=-=-=-==-
Scotts Contracting offers Green Building Services for the St Louis Metro Area
Click here to email Scotty to schedule a Free Green Site Evaluation
-- Scott's Contracting scottscontracting@gmail.com http://www.stlouisrenewableenergy.blogspot.com http://www.stlouisrenewableenergy.com scotty@stlouisrenewableenergy.com6.28.2010
The Energy in Our Future- Where will it Come From?
The Energy in Our Future- Where will it Come From: Oil, Coal, Natural Gas, Solar, Wind, Geo Thermal? Utilize the following resources and make your Best Guess.
My Guess is:
a) Energy Costs will Continue to Rise because access to Oil is becoming harder to locate and produce.
b) Coal and the process to turn it into Energy is Inefficient and Harmful to the environment.
c) Politics within the USA will Keep the Renewable Energy Resources from becoming Main Stream and affordable to the masses.
Feel Free to post your Ideas and Solutions in the Comments Section to follow the Article. If you would like your Ideas Published send them to scotty@stlouisrenewableenergy.com.________________________________________
The Fake Fire Brigade - How We Cheat Ourselves about our Energy Future
A comment to begin with: IIER is a research organization trying to neutrally assess the situation of our societies, and with that, find out what strategies work and which ones do not. By no means are we trying to promote or discourage any specific energy alternative, and we have no vested interest in anything else than stable future energy supplies. What you read below is the result of years of thorough analysis and research. When we began, we were completely neutral towards any particular solution and technology, and our only aim was to understand the implications of various energy scenarios on the future of our societies. Now, we have an opinion.
The longer straw - the future of fossil fuels (and most other resources)
The future of fossil fuels, particularly of oil, but also many other resources including water and minerals, looks problematic. People keep discussing proven reserves and whether peak oil already has arrived or not. Unfortunately, we will only be able to put this argument to rest in hindsight. But what is more important is the fact that - no matter how much additional oil we can still retrieve - future barrels will be much more difficult to extract relative to the past.
Drilling a hole in the desert and waiting for black gold to gush out is infinitely less complex than drilling a much deeper hole 5000 feet under water, as the public is now painfully beginning to understand. Many experts agree that we probably have used about 40-50% of recoverable oil. It is difficult to prove such numbers, but we may for a minute assume that this is true. For pessimists, this makes our glass half empty. For optimists, it remains half full. This has been the exact argument the energy community has been having, to little avail, so let's play with that analogy some more: our oil reserves can be compared with a 1 mile deep glass full of our favorite drink. Getting the first sips is easy. Whenever we are thirsty, we lower a straw into the fluid and drink as much as we like. After a while, that straw might become too short, so we have to find a longer one. Not really a problem. We might even get better at making straws for a while. And so it continues.
But once we are half a mile down into this huge glass, the straw will be so long that one might need help to even hold it, and we will most likely require help to suck hard enough to make the fluid come all the way up. What has happened? We still have half of our favorite drink left, but the efforts to get to it are becoming increasingly painful, significantly diminishing the net benefit of that next sip. And so we might (have to) give up drinking long before the glass is empty, just because its too difficult to get at the fluid in a meaningful way, and because the effort of sucking eventually exceeds the benefit and joy from each sip.
The concept behind our "mile-long glass" analogy unfortunately applies to almost every raw material and energy source we are currently using. The more we have extracted, the more difficult it becomes to get to the next unit. Our organization (IIER) looks at this phenomenon using the term "Resource Return on Energy Investment" (RREI), which is based on established approaches used for Energy Returns on (Energy) Investment (EROI). It describes the amount of effort (energy) needed to get one unit of a resource we want to extract. To extract the next unit, our effort typically increases compared to the past, as we have mostly exploited the easy finds and must pursue the ones that are further away, harder to get, more difficult to secure politically, or any such combination. Over time, this increasing effort makes the production less and less useful to societies. Or to use our drinking straw example: at one point sucking out more from that glass exhausts us so much (e.g. the energy invested per sip becomes so big) that we will have to stop our effort and turn to something else, or - if there is no equivalent alternative - drink less.
When looking at RREI, almost all resources currently used in human processes show declines. Less "easy oil" means that we have to drill in hostile environments deep under the surface of oceans, lower ore grades mean that we have to move four times as much rock to extract the same amount of copper when compared to a couple of decades ago, and the depletion of groundwater sources translates to getting drinking water from desalination plants or from fossil (non-renewable) aquifers far away, at much higher energy cost.
This decline in easily extractable resources and the increased effort to retrieve them is much more important than the exact year when peak production of a particular resource actually occurs. It is today's reality, and helps explain why we are drilling at the bottom of the ocean at depths where no human being could survive for even a second.
Renewable energies - the fake fire brigade
So while some haven't really recognized that we will soon run into serious problems from traditional fossil fuels, others are already preparing a "brighter future", which will bring independence from coal, oil and gas, with far lower carbon dioxide emissions to boot. In many European countries, thanks to subsidies and purchasing guarantees, large amounts of renewable electricity generation capacity has been built during the last decade, and in the U.S., corn based ethanol now has a government-mandated share of up to 10% in fuel gasoline. But let us not fool ourselves: during those 10 years, despite all the relative successes, renewable energies (including hydropower) grew by far less compared to the global increase in total energy consumption. Overall, our global energy delivery system continues to be as dependent on fossil fuels as ever before, or even more so. On top of that, even those renewable technologies are mostly based on fossil fuel inputs, which are either used during the manufacturing of the equipment, or even during production and processing (e.g. biofuels).
One of IIER's key objectives is to understand what the future of energy delivery systems will look like. We know that we will have to face a future with less and ultimately no fossil fuels. The question remains how to prepare for this eventuality. Most technological optimists believe that this challenge can be met with some combination of biofuels, renewable electricity generation technologies, electric cars, smart grids, and many other investments. However, when we examine these technologies more closely, none of these so-called "solutions" come close to providing any relief, quite the contrary.
As Robert Rapier, a well-respected energy analyst, puts it: "We are running out of traditional energy sources, which can be compared to our house being on fire. While that happens, many people linger around the burning building and pretend to be firemen, mimicking their actions, carrying some equipment, shouting commands - but actually they have no real water, no real skills, no appropriate tools. That way your house will burn to the ground because the "real" firemen never showed up, as everybody thinks there are more than enough firemen on site." This is exactly what it is: when taking a closer look, most - almost all - of the renewable energy technologies promoted today won't solve any of our future energy problems. Let's get into the details using two examples. Renewable electricity generation, and biofuels. In order to keep this article halfway short, we will only make the general case, but we are happy to back up our claims with hard facts.
The future of electricity – a shaky one
Today's electricity grids are key building blocks of modern civilizations. Advanced economies depend on the reliable and discretionary delivery of power to every single socket. Our way of living, which includes the ability to read this article, wouldn't be possible without. Unfortunately, delivering stable electricity poses a significant challenge to grid operators, as energy production and consumption in any moment need to be matched explicitly. Storage is expensive, technologically complex, and always incurs losses, which is why power grids have become the perfect example of just-in-time supply chains. Whenever there is growing demand, additional power generation capacity comes online within seconds, and likewise, falling demand leads to the immediate withdrawal of an equal amount of generation capacity.
Having access to stable power grids seems to be positively correlated with economic output as IIER's EAI (Electricity Availability Index) shows. It is based on availability (percent of population with access to electricity) and reliability (number and duration of blackouts). When looking at the chart, it becomes obvious that it seems almost impossible for a country to arrive at a per-capita GDP significantly above US$ 10'000 (2007 dollars, adjusted for purchasing power parity) in environments where electricity isn't a stable and reliable commodity. When thinking about it, this isn't so surprising, as most industrial and commercial processes require stable electricity in large quantities, and its absence simply makes many things impossible.
Right now, all our electricity delivery systems are almost fully controlled from the supply-side, i.e. no usage restrictions apply, which is why we benefit so much. Customers don't have to pre-order a certain amount of electricity before they can turn on a machine, a computer, or start cooking, but instead just do so, mostly oblivious to the fact that someone somewhere in a grid operations center will turn on a gas turbine, or let some water flow downstream, just because we flip a switch. A preliminary analysis conducted by IIER shows that less than 10% of electricity demand can theoretically be supply-controlled without severely impacting societies. Computers, machines, air conditioners, stoves and ovens, and most other industrial and household devices are those things we want to use when we need them. But even where grid operators theoretically could shift certain electricity uses to off-peak times without disrupting our lives, this comes at the significant price of introducing a smart grid infrastructure, and new devices capable of being controlled remotely. Another fake fireman.
Thus, no matter how hard we try, electricity systems will continue to rely mostly on supply side adjustments. Today, this is manageable, because most sources are either providing steady power flows (such as coal, nuclear or run-of-river hydro power plants) or then they are mostly controllable (such as gas fired power plants or hydropower from dammed water pools). With that mix of inputs, electricity on demand becomes possible for most advanced economies. Additions of wind and solar power over the last decade introduced renewable electricity generation technologies into the grid. Those two sources have none of the above qualities: they neither provide steady flows, nor are they controllable. "No wind" means "no power", so does "no sunshine", and even sharing across long-distances using high voltage DC (HVDC) transmission lines won't change that fact, due to the stochastic nature of the inputs. Potentially crippling power outages will happen regularly in societies that rely on large percentages of these technologies to meet their electricity demand. With that, the current system of just-in-time electricity delivery would be replaced by one with irregular service interruptions. And yet there are plans made worldwide suggesting that we can produce 20, 30 or 50% of our future electricity consumption from those two sources. This is self-deception at best, and a lie at worst, as it is simply impossible to manage delivery systems where both inputs and outputs are largely uncontrollable, irrespective of other features added.
What is important here: we're not talking about a future where renewable energies supplement fossil fuel based electricity systems like they do today. Given sufficient backup generation systems powered by fossil fuels, a larger penetration of renewable electricity is definitely possible, and might reduce carbon dioxide production and other externalities, albeit at a horribly high cost. However, these types of add-in systems fail to break our dependence on fossil fuels and don't prove that we can deliver stable electricity in a world where renewable sources supply a majority of inputs into electricity grids. If that was the objective, we should be honest and just build some wind turbines and match them with gas fired generation capacity for low-wind times, instead of talking about long distance transmission, smart grids, and other technologies that despite their cost don't have the potential to secure the basic objective: stable power at any time.
Someone in the renewable electricity world would probably argue that this is where storage can play an important role. Unfortunately, again, this is more self-deception. Right now, storage that balances renewable sources comes from the flexibility of other stock-based supplies, such as natural gas and hydropower. They can be turned off when the wind blows, and turned on when it stops. The reason why this works is because renewables have such a small market share and often use much larger backup systems. Denmark for example operates its heavily wind-based electricity system with the backing of comparably huge hydro power plants in Norway and Sweden, an approach which unfortunately isn't scalable globally. Not many countries have neighbors with flexible energy generation capacity ten times their own, and that is about what is needed to buffer the huge long-term variability of renewable electricity generation.
So let's for a minute assume that the United Kingdom - one of the world's "best" places to generate electricity from wind - runs on 20% wind power as planned in the least ambitious scenarios currently promoted, and that standby natural gas power plants become no longer available to bridge supply gaps. Some say that ELVs (electric cars) could provide the necessary storage capacity. We did the maths: the total annual output from wind in a 20% scenario for England and Wales would amount to approximately 64 TWh (20% of total current demand). After modeling a nationwide wind turbine network using the best 50 locations (we even included Scotland), we calculated the necessary storage to bridge the largest possible supply gap (e.g. when the wind doesn't blow for a number of days) and found that Britain in 2009 would have needed 96.5 million battery operated electric cars with 40 kWh batteries each fully available for storage, e.g. no longer ready to be driven. For comparison: 28.5 million private vehicles are currently registered in the UK. The problem here is that wind patterns don't just include short term ups and downs, but instead do involve long periods with very little wind, and then long periods with a lot. Unfortunately, this pattern isn't even predictable year-over-year. Buffering those resources is not something that can be managed with storage, no matter how large. Another fake fireman.
The truth about electricity is simple, surprising and daunting: with the most promising renewable technologies - wind and solar - irrespective of expensive supplements being added, electricity systems as we know them today will not be able to operate. But instead of putting efforts towards finding real solutions, we are spending billions, likely even trillions, of dollars and Euros on technologies that cannot and will not work in the way we expect them to. Again, as a reminder: this is not an argument to defend the way we currently produce electricity, but a strong encouragement to research how we might get reliable power to our ubiquitous sockets without fossil fuels providing the major part. And for those who now suggest to go for a nuclear option: irrespective of any argument about long-term risk, this technology too has a number of downsides, among them the inability to control output according to demand, relatively high cost, and a high dependence on fossil fuels both for the construction of plants and the mining of uranium. And last, but not least, the fact that uranium too, is a non-renewable resources, subject to the fact that we will eventually arrive at the limits of meaningfully extractable material (e.g. the ones offering an attractive RREI) - particularly if we plan on scaling up nuclear power to replace other fuels.
Combustion fuels – headaches all over
The other big challenge ahead lies in fuels used for transportation and heating, mainly in oil. This is the place where scarcity is most apparent, as described above. We wouldn't try to drill in deep water or extract oil from shales if it wasn't for the inability to find and explore easier and cheaper sources. What this has done, at a minimum, is lifted the cost of oil to above 70 US$ a barrel, about three times its inflation-adjusted long-term average price. This is not because of speculation, as some claim, but just because it costs 60-70 US$ to extract those least attractive sources. Thus, we truly have to start thinking about alternative ways to move our cars, trucks, planes and even tractors.
The easy way out would be electric vehicles, but after reading the above paragraph on electricity, this might not be an entirely safe bet. And that doesn't even take into account the still existing technology and cost problems with battery technology.
One of the many challenges of a number of renewable energy technologies is that they are themselves heavily dependent on fossil fuel inputs. This is true for raw material extraction and manufacturing of solar panels, wind turbines and other things, but even more so for many so called "green" fuels. Significant inputs to the production process of biofuels - for example of corn based ethanol - come in the form of oil (fuels, pesticides), coal (electricity) or natural gas (fertilizer). This has two consequences: first, it doesn't break our dependence from fossil fuels and second, as fossil fuels become more expensive, so do these "alternatives".
However, one of the biggest challenges of all renewable (green) fuels is their limited availability. There simply isn't enough biomass potential in any Western society to produce a sufficient amount of non-fossil combustion fuels that could meaningfully replace what fossil fuels we use.
Brazil, which is often used as the poster-child of biofuels production and use, provides a stark reminder that building an oil-independent society with biomass-based transportation fuels is nothing but a dream. In 2008, Brazil produced (and mostly consumed) approximately 163.5 million barrels of ethanol. In the same year, the country consumed 907 million barrels of crude oil. Given the lower energy content in ethanol (3.53 MBtu per barrel vs. 5.8 MBtu for oil), biofuels had a share of not more than 9.9% of the two, while crude oil provided 90.1% of the total energy in liquid fuels. So much for Brazil running on renewable biomass. But that is just the beginning: when taking into account that Brazil is an emerging economy, and one of the least densely populated countries, the problem becomes even more obvious. With approximately 0.51 BOE (barrels of oil equivalent) per capita, the U.S. produced about exactly as much biofuel per person as Brazil did (0.52 BOE/capita). The only difference was that overall consumption of oil and biofuels together was 4.6 times larger in the U.S. when compared to Brazil, and twice as large in Europe (EU-27). Europe however, has yet another handicap limiting its ability to go for biomass. It has about 3.6 times as many people per square kilometer than the U.S., and about 5 times as many as Brazil, which constrains the continent's ability to grow enough biomass for biofuels and feed all its people at the same time.
So in a nutshell, there is no such thing as a replacement for fossil liquids coming from biofuels, instead this is just one more of those fake fire brigades.
A true plan for the future – begin from the other end
All of today's planning efforts take place based on today's energy delivery systems. We add some renewables to the current mix and see how we can manage. When we see that this causes problems, we respond by adding highly complex and costly bells and whistles. Alternatively, we start introducing new technologies that will never be able to truly scale up, are in fact heavily dependent on fossil fuel inputs, or both. We would go so far as to say that we can safely prove that more than 90% of energy system alternatives discussed and introduced today have no potential of helping us to secure a longer term energy future.
We are thus not sure if it is a good idea to put all of society's efforts into fixes and add-ons to today's energy delivery and consumption systems, but instead we strongly recommend the development of approaches and technologies that radically break with a fossil fuel base. The only meaningful way of looking at the future of energy delivery and application technologies would be to build energy systems based on an assumption that renewable technologies have to provide the entire amount required by our societies, and then to reshape societies so they are in line with what and how these technologies can deliver.
Only when applying this (what is probably considered radical) view, we would be able to model a sustainable and reliable energy future. Once we have figured out how this can work, we may still consider how to make the best use of our remaining fossil fuels, but going the other way will just fool us into believing that we have solutions, until we recognize we don't. And today, to be frank, this is exactly where we are. A lot of fake firemen are standing around a fire that is right now openly breaking out.
IIER puts substantial effort into trying to understand what energy systems could work in the long run. But unfortunately, very few other people do so, which is something we want to change. Instead of spending billions or even trillions on amendments that most likely won't help, a significant portion of these investments should go into a completely new design of our energy future. Let's finally bring in the real fire brigade.
Resources and links
Editor's note: Above Post: is a guest post from Hannes Kunz, President of Institute for Integrated Economic Research (IIER). Hannes has a PhD in Economics from St. Gallen University and resides in Zurich Switzerland. IIER, is a non-profit organization that integrates research from three different areas: the financial/economic system, energy and natural resources, and human behavior. Their objective is to aid policymakers in developing strategies that result in more benign trajectories after global growth ends. Hannes is also a friend and co-author of two papers with me, (pub. pending), 'Net Energy and Time', and 'Net Energy and Variability'.
St Louis Renewable Feed
Featured Post
How Two Friends Turned Abandoned CASTLE into a 4☆HOTEL | by @chateaudut...
Join us on an extraordinary journey as two lifelong friends, Francis and Benoit, turn a crumbling, centuries-old castle into a stunning 4-st...
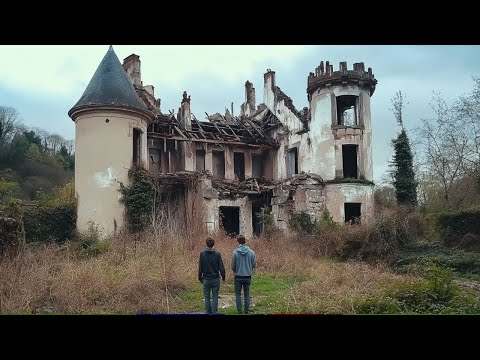
-
Thank You for stopping by the Green Blog. If additional information in needed or you have a question let me know by posting a question or ...
-
Convection is the movement of air in response to heat Warm air rises, cool air sinks. Because walls and windows are usually cooler than th...
-
Green Building Priority #6 – Ensure Durability Number 6 in my list of the top-10 green building priorities is to ensure that the home is dur...